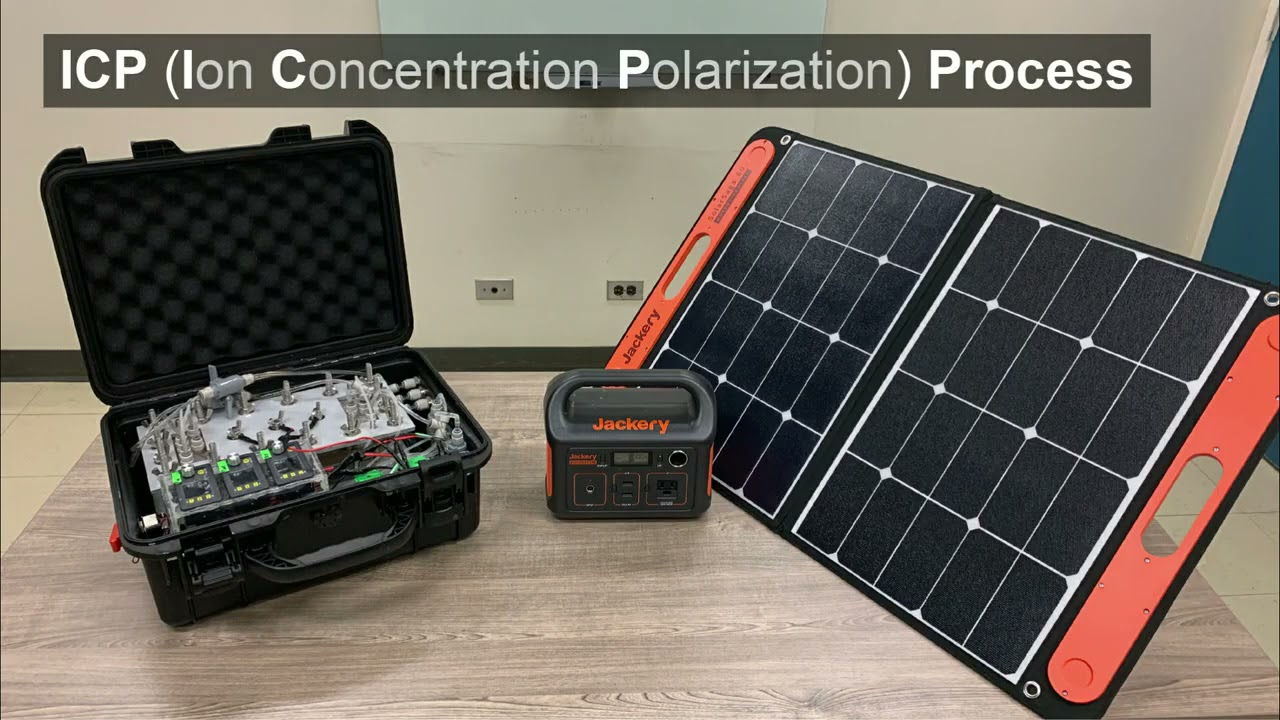
From Seawater to Drinking Water at the Push of a Button – With No Filters!
Portable Seawater Desalination System for Generating Drinkable Water in Remote Locations
Abstract
A portable seawater desalination system would be highly desirable to solve water challenges in rural areas and disaster situations
While many reverse osmosis-based portable desalination systems are already available commercially, they are not adequate for providing reliable drinking water in remote locations due to the requirement of high-pressure pumping and repeated maintenance. We demonstrate a field-deployable desalination system with multistage electromembrane processes, composed of two-stage ion concentration polarization and one-stage electrodialysis, to convert brackish water and seawater to drinkable water. A data-driven predictive model is used to optimize the multistage configuration, and the model predictions show good agreement with the experimental results.
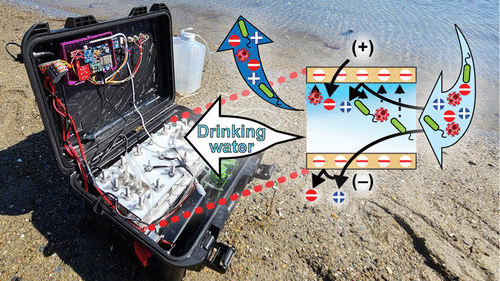
The user-friendly unit, which weighs less than 10 kilograms and does not require the use of filters, can be powered by a small, portable solar panel. Credit: M. Scott Brauer
Researchers build a portable desalination unit that generates clear, clean drinking water without the need for filters or high-pressure pumpsMIT researchers have developed a portable desalination unit, weighing less than 10 kilograms (22 pounds), that can remove particles and salts to generate fresh drinking water.
The device, which is about the size of a suitcase, needs less power to operate than a cell phone charger. It can also be driven by a small, portable solar panel, which can be purchased online for around $50. It automatically generates drinking water that exceeds World Health Organization (WHO) quality standards. The technology is packaged into a user-friendly device that runs with the push of a single button.
Unlike other portable desalination devices that require water to pass through filters, this unit utilizes electrical power to remove particles from drinking water. Eliminating the need for replacement filters significantly reduces the long-term maintenance requirements.
The setup includes a two-stage ion concentration polarization (ICP) process, with water flowing through six modules in the first stage then through three in the second stage, followed by a single electrodialysis process Credit: M. Scott Brauer
This could enable the unit to be deployed in remote and severely resource-limited areas, such as communities on small islands or aboard seafaring cargo ships. It could also be used to aid refugees fleeing natural disasters or by soldiers carrying out long-term military operations.
“This is really the culmination of a 10-year journey that I and my group have been on. We worked for years on the physics behind individual desalination processes, but pushing all those advances into a box, building a system, and demonstrating it in the ocean, that was a really meaningful and rewarding experience for me,” says senior author Jongyoon Han, a professor of electrical engineering and computer science and of biological engineering, and a member of the Research Laboratory of Electronics (RLE).
Joining Han on the paper are first author Junghyo Yoon, a research scientist in RLE; Hyukjin J. Kwon, a former postdoc; SungKu Kang, a postdoc at Northeastern University; and Eric Brack of the U.S. Army Combat Capabilities Development Command (DEVCOM). The research has been published online in the journal Environmental Science and Technology.
Filter-free technologyCommercially available portable desalination units typically require high-pressure pumps to push water through filters, which are very difficult to miniaturize without compromising the energy-efficiency of the device, explains Yoon.
Instead, their unit relies on a technique called ion concentration polarization (ICP), which was pioneered by Han’s group more than 10 years ago. Rather than filtering water, the ICP process applies an electrical field to membranes placed above and below a channel of water. The membranes repel positively or negatively charged particles — including salt molecules, bacteria, and viruses — as they flow past. The charged particles are funneled into a second stream of water that is eventually discharged.
The process removes both dissolved and suspended solids, allowing clean water to pass through the channel. Since it only requires a low-pressure pump, ICP uses less energy than other techniques.
The portable device does not require any replacement filters, which greatly reduces the long-term maintenance requirements. Credit: M. Scott Brauer
But ICP does not always remove all the salts floating in the middle of the channel. So the researchers incorporated a second process, known as electrodialysis, to remove remaining salt ions.
Yoon and Kang used machine learning to find the ideal combination of ICP and electrodialysis modules. The optimal setup includes a two-stage ICP process, with water flowing through six modules in the first stage then through three in the second stage, followed by a single electrodialysis process. This minimized energy usage while ensuring the process remains self-cleaning.
“While it is true that some charged particles could be captured on the ion exchange membrane, if they get trapped, we just reverse the polarity of the electric field and the charged particles can be easily removed,” Yoon explains
They shrunk and stacked the ICP and electrodialysis modules to improve their energy efficiency and enable them to fit inside a portable device. The researchers designed the device for nonexperts, with just one button to launch the automatic desalination and purification process. Once the salinity level and the number of particles decrease to specific thresholds, the device notifies the user that the water is drinkable.
The researchers also created a smartphone app that can control the unit wirelessly and report real-time data on power consumption and water salinity.
Beach testsAfter running lab experiments using water with different salinity and turbidity (cloudiness) levels, they field-tested the device at Boston’s Carson Beach.
Yoon and Kwon set the box near the shore and tossed the feed tube into the water. In about half an hour, the device had filled a plastic drinking cup with clear, drinkable water.
“It was successful even in its first run, which was quite exciting and surprising. But I think the main reason we were successful is the accumulation of all these little advances that we made along the way,” Han says.
The resulting water exceeded World Health Organization quality guidelines, and the unit reduced the amount of suspended solids by at least a factor of 10. Their prototype generates drinking water at a rate of 0.3 liters per hour, and requires only 20 watts of power per liter.
“Right now, we are pushing our research to scale up that production rate,” Yoon says.
One of the biggest challenges of designing the portable system was engineering an intuitive device that could be used by anyone, Han says. Yoon hopes to make the device more user-friendly and improve its energy efficiency and production rate through a startup he plans to launch to commercialize the technology.
In the lab, Han wants to apply the lessons he’s learned over the past decade to water-quality issues that go beyond desalination, such as rapidly detecting contaminants in drinking water.
“This is definitely an exciting project, and I am proud of the progress we have made so far, but there is still a lot of work to do,” he says.
For example, while the “development of portable systems using electro-membrane processes is an original and exciting direction in off-grid, small-scale desalination,” the effects of fouling, especially if the water has high turbidity, could significantly increase maintenance requirements and energy costs, notes Nidal Hilal, professor of engineering and director of the New York University Abu Dhabi Water research center, who was not involved with this research.
“Another limitation is the use of expensive materials,” he adds. “It would be interesting to see similar systems with low-cost materials in place.”
Reference: “Portable Seawater Desalination System for Generating Drinkable Water in Remote Locations” by Junghyo Yoon, Hyukjin J. Kwon, SungKu Kang, Eric Brack and Jongyoon Han, 14 April 2022, Environmental Science and Technology. DOI: 10.1021/acs.est.1c08466
The research was funded, in part, by the DEVCOM Soldier Center, the Abdul Latif Jameel Water and Food Systems Lab (J-WAFS), the Experimental AI Postdoc Fellowship Program of Northeastern University, and the Roux AI Institute.